Process of Science
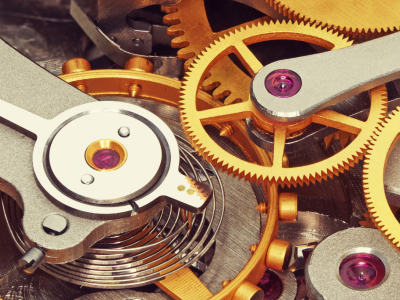
The Culture of Science
Ideas in Science
Research Methods
- The Practice of Science: An introduction to research methods
- Experimentation in Scientific Research: Variables and controls in practice
- Description in Scientific Research: Observations and multiple working hypotheses
- Comparison in Scientific Research: Uncovering statistically significant relationships
- Modeling in Scientific Research: Simplifying a system to make predictions
Data
Scientific Communication
- Understanding Scientific Journals and Articles: How to approach reading journal articles
- Utilizing the Scientific Literature: The record of scientific progress
- Peer Review in Scientific Publishing: What it is and how it works
- The How and Why of Scientific Meetings: How to approach attending a meeting
Sign in or register
For an ad-free experience and access the Visionlearning Classroom, sign in or register.