Atomic Theory I: Detecting electrons and the nucleus
by Adrian Dingle, B.Sc., Anthony Carpi, Ph.D.
Listen to this reading
00:00Did you know that for 100 years scientists believed that atoms were the smallest particles that existed? It took many scientists and numerous experiments to show that atoms were made up of smaller particles with very different properties. Over a 75-year period beginning in the first part of the 19th century, two subatomic particles were discovered: the electron and the nucleus. In addition, by means of a clever experiment the negative charge of a single electron was calculated.
Atoms are not dense spheres but consist of smaller particles including the negatively charged electron.
The research on passing electrical currents through vacuum tubes by Faraday, Geissler, Crookes, and others laid the groundwork for discovery of the first subatomic particle.
J.J. Thomson’s observations of cathode rays provide the basis for the discovery of the electron.
Rutherford, Geiger, and Marsden performed a series of gold foil experiments that indicated that atoms have small, dense, positively-charged centers – later named the nucleus.
Millikan’s oil drop experiment determines the fundamental charge on the electron as 1.60 x 10-19 coulombs.
This module is an updated version of Atomic Theory I.
By the late 1800’s, John Dalton’s view of atoms as the smallest particles that made up all matter had held sway for about 100 years, but that idea was about to be challenged. Several scientists working on atomic models found that atoms were not the smallest possible particles that made up matter, and that different parts of the atom had very distinct characteristics.
Faraday’s observations
The English scientist Michael Faraday can reasonably be considered one of the greatest minds ever in the fields of electrochemistry and electromagnetism. Somewhat paradoxically, all of Faraday’s pioneering work was carried out prior to the discovery of the fundamental particle that these electrical phenomena depend upon. However, one of Faraday’s earliest experimental observations was a crucial precursor to the discovery of the first subatomic particle, the electron.
As early as the mid-17th century, scientists had been experimenting with glass tubes filled with what was known then as rarefied air. Rarefied air referred to a system in which most of the gaseous atoms had been removed, but where the vacuum was not complete. In 1838, Faraday noted that when passing a current through such a tube, an arc of electricity was observed. The arc started at the negative plate (known as the cathode) and traveled through the tube to the oppositely charged anode (Faraday, 1838).
In his experiments, Faraday observed a luminescence that started part way down the tube, and traveled toward the anode. This left an area between the cathode and the start of the luminescence that was not illuminated, and subsequently became known as Faraday’s dark space (Figure 1). Faraday couldn’t fully explain his observations, and it took a number of further developments in terms of the technology of the tubes, before a greater understanding emerged.
Figure 1: Glow discharge in a low-pressure tube caused by electric current. Like what Faraday saw, the tube shows a dark space between the glows around the cathode (left, negatively charged) and anode (right, positively charged).
image ©Andrejdam/WikimediaComprehension Checkpoint
Advancing technology moves science forward
In 1857 the German glassblower Heinrich Geissler, while working for fellow countryman and physicist Julius Plücker at the University of Bonn, improved the quality of the vacuum that could be achieved in such tubes. However, Geissler’s tubes still contained enough gaseous atoms that when the electrical current travelled in the tube, there was an interaction between the two, causing the tubes to glow. In the mid-19th century these Geissler tubes were largely nothing more than a curiosity, but interestingly, a curiosity that proved to be a forerunner of neon lights.
Englishman William Crookes repeated experiments similar to those of Faraday and Geissler, but this time with the ‘new and improved’ vacuums. The number of gas atoms (and hence the pressure) was drastically reduced in Crookes’ tubes. This caused an interesting effect: Faraday’s dark space was observed even further down the tube, again extending away from cathode toward the anode.
In addition to the extension of the dark space, fluorescence was observed on the glass behind the anode at the positive end of the tube. When further experimentation revealed that shadows of objects placed in the tube were cast onto the glass behind the anode, the German physicist Johann Hittorf proposed that the shadows must have been created by something travelling in a straight line from the cathode to the anode. Yet another German physicist Eugen Goldstein, christened these invisible beams cathode rays.
J.J. Thomson and the first subatomic particle
As discussed in our module Early Ideas about Matter, Dalton’s atomic theory suggested that the atom was indivisible, i.e., that it was the smallest particle that made up matter, and that all matter was based upon that single unit. Experiments with cathode ray tubes dramatically changed that view when they led to the discovery of the first subatomic particle.
J.J. Thomson was an English physicist who worked with cathode ray tubes similar to those used by Crookes and others in the mid-19th century. Thomson’s experiments (Thomson, 1897) went further than those before him and provided evidence of the properties of the “something” hinted at by Hittorf. Thomson noted that cathode rays were deflected by magnetic fields and that the deflection was the same no matter what the source of the rays. This suggested that the rays were universal in their properties and that they had some magnetic charge. Thomson further demonstrated that cathode rays were charged because they could be deflected by an electric field. He found that the rays were deflected toward a positive plate and away from a negative plate, thus determining that they were made up of negatively charged particles of some sort.
Finally, Thomson applied both electrical and magnetic fields to the cathode ray at the same time. Knowing the strength of the fields applied and measuring the deflection of the particle stream in the tube, he was first able to measure the velocity of the particles in the stream. Then, by measuring the deflection of the ray while varying the two fields, Thomson was able to measure the mass-to-charge ratio of the particles in the stream and he found something astonishing. The negative particles had a mass-to-charge ratio that was over 1,000 times lower than that of a hydrogen atom, suggesting that the particles were incredibly tiny – much smaller than the smallest atom known. This fact allowed Thomson to definitively say that the atom was not the fundamental building block of matter and that smaller (subatomic) particles existed. Thomson originally called these particles corpuscles, but later they became known as electrons.
Comprehension Checkpoint
The electron explains so much
Thomson’s discovery made sense of all of the previous observations made by Faraday, Geissler, and Crookes. Zipping through a tube filled with gas but partially under vacuum, electrons would eventually slam into those gas atoms, knocking off some of their electrons and making them fluoresce. The dark space that Faraday first noted was due to the distance needed for the electrons to accelerate to the speed necessary to ionize the tube’s gas atoms.
In the better vacuums achieved in the Crookes’ tubes, the electrons could travel further distances without interacting with gas molecules because of the lower density of molecules in the tube, thus extending the dark space.
Comprehension Checkpoint
The ‘Plum Pudding’ model
With the electron now discovered, Thomson went on to propose an entirely new model of the atom that was known as "The Plum Pudding Model." The model was so called since it mimicked the British desert of the same name that had dried fruit (primarily raisins not plums), dispersed in a body of suet and eggs that made a dough.
In his model Thomson proposed that the negatively charged electrons (analogous to the raisins) were randomly spread out among what he called "a sphere of uniform positive electrification" (analogous with the dough or body of the pudding) (see Figure 2).
Figure 2: Thomson's "plum pudding model" of the atom, showing a positively-charged sphere containing many negatively-charged electrons in a random arrangement.
Thompson’s model of the atom as a doughy clump of positive and negative particles persisted until 1911, when Ernest Rutherford, a former student of Thomson’s, advanced atomic theory yet another notch.
Rutherford’s gold foil experiment and the nucleus
During the years 1908–1911, Ernest Marsden and Hans Geiger performed a series of experiments under the direction of Ernest Rutherford at the University of Manchester in England. In these experiments alpha particles (tiny, positively charged particles) were fired at a thin piece of gold foil (Figure 3). Under the Thomson Plum Pudding model of the atom, the "sphere of uniform positive electrification" was thought to be so diffuse that the tiny, fast moving alpha particles would pass straight through. Similarly, the electrons in the model were thought to be so tiny that any electrostatic interactions between them and the positive alpha particles would be minimal, so the path of the alpha particles would hardly be affected.
Figure 3: The gold foil experiment designed by Rutherford, Marsden, and Geiger. A beam of positively charged alpha particles was shot at a piece of gold foil. A screen around the foil captured the impact of the alpha particles.
As predicted, Rutherford and his co-workers observed that most of the alpha particles passed straight through the gold foil, and some particles were deflected at small angles. However, contradictory to what the Plum Pudding model predicted, a few rebounded at very sharp angles, some even flying straight back toward the source! These particles were acting as if they were encountering a hard object, like a tennis ball bouncing off a brick wall (Figure 4).
Figure 4: In the gold foil experiment, Rutherford and his colleagues expected to see the alpha particles passing through the mostly empty "Plum Pudding"-style atoms. However, what they observed was that the alpha particles occasionally ricocheted at sharp angles, indicating there was something more solid in the atom than previously thought.
The fact that most of the alpha particles passed straight through the gold foil suggested to Rutherford that atoms are made up of largely empty space. However, contrary to the Thomson Plum Pudding model, Rutherford’s work suggested that there was a dense, positively charged area in an atom that caused the observed repulsion and backscattering of alpha particles. Rutherford was astonished by these observations and famously said:
It was quite the most incredible event that has ever happened to me in my life. It was almost as incredible as if you fired a 15-inch shell at a piece of tissue paper and it came back and hit you. On consideration, I realized that this scattering backward must be the result of a single collision, and when I made calculations I saw that it was impossible to get anything of that order of magnitude unless you took a system in which the greater part of the mass of the atom was concentrated in a minute nucleus. It was then that I had the idea of an atom with a minute massive centre, carrying a charge.
Over a series of experiments and papers (Rutherford, 1911, 1913, 1914), Rutherford developed a model of the atom with a dense, positively charged area of the atom at the center, now known as the nucleus – and the nuclear model of the atom was born.
Comprehension Checkpoint
Millikan and the specific charge on the electron
Following the discovery of the electron, Nobel Prize-winning physicist Robert Millikan conducted an ingenious experiment that allowed for the specific value of the negative charge of the electron to be calculated. In his famous oil drop experiment, Millikan and co-workers sprayed tiny oil droplets from an atomizer into a sealed chamber (Millikan, 1913). The oil drops fell downward, under the influence of gravity, into a space between two electrical plates. There they became charged, by interacting with air that had been ionized by X-rays.
Figure 5: Millikan's oil drop experiment in which he observed droplets of oil fall between two electrical plates, where the droplets became ionized by X-rays.
By adjusting the voltage between the two electrical plates, Millikan applied an electrical force upward that exactly matched the gravitational force downward, thus suspending the drops motionless. When suspended, the electrical force and the force of gravity were working in opposite directions but were equal in magnitude. Hence:
where q is the charge on the oil drop, E is the electric field, m is the mass of the oil drop, and g is the gravitational field. By measuring the mass of each oil drop and knowing both the gravitational and the electrical field, the charge on each drop could be determined.
Millikan found that there were differing charges on different oil drops. However, in each case the charges on the oil drops were found to be multiples of 1.60 x 10-19 coulombs. He concluded that the differing charges were due to different numbers of electrons, each having a negative charge of 1.60 x 10-19 coulombs, and hence the charge on the electron was found.
Comprehension Checkpoint
Another step toward a theory of the atom
Thomson’s electron and Rutherford’s nuclear model were tremendous advancements. The Japanese scientist Hantaro Nagaoka had previously rejected Thomson’s Plum Pudding model on the grounds that opposing charges could not penetrate each other, and he counter-proposed a model of the atom that resembled the planet Saturn with rings of electrons revolving around a positive center. Upon hearing of Rutherford’s work, he wrote to him in 1911 saying, "Congratulations on the simpleness of the apparatus you employ and the brilliant results you obtained."
But, the planetary model was not perfect, and several inconsistent experimental observations meant much work was still to be done. At the time the electron was still thought of as a small particle, and it was thought to spin almost randomly around the nucleus of the atom. It would take the additional experiments and the genius of Neils Bohr, Max Planck, and others to make the paradigm shift from classical physics in which atoms consist of tiny particles and are governed by laws of motion, to quantum mechanics in which electrons behave like waves and exhibit strange and exotic behaviors. (See our interactive animation comparing orbital and quantum models of the first 12 elements.)
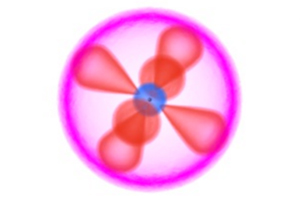
Interactive Animation: Atomic and ionic structure of the first 12 elements
Table of Contents
- Faraday’s observations
- Advancing technology moves science forward
- J.J. Thomson and the first subatomic particle
- The electron explains so much
- The ‘Plum Pudding’ model
- Rutherford’s gold foil experiment and the nucleus
- Millikan and the specific charge on the electron
- Another step toward a theory of the atom
Activate glossary term highlighting to easily identify key terms within the module. Once highlighted, you can click on these terms to view their definitions.
Activate NGSS annotations to easily identify NGSS standards within the module. Once highlighted, you can click on them to view these standards.